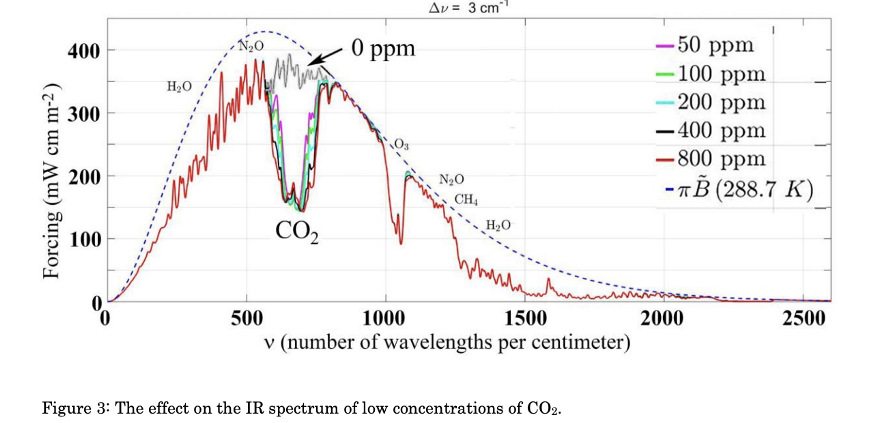
Prof. Kees de Lange met William Happer during his visit in The Netherlands last November. After that they exchanged several emails about the paper Happer en his colleague William van Wijngaarden wrote. De Lange kindly translated his knowledge of the paper into a blog article that is non-technical.
This is an excellent paper that the authors qualify as a review article, but which has much more to offer. It is particularly well written, leads the reader through the complicated maze of relevant atmospheric physics, and has a lot of new science to offer. The paper is long, but for very good reasons.
The atmosphere of planet Earth is a very complex system. To describe such a system in terms of sophisticated physics is a major task. The good news is that much of modern day physics is applicable. On the other hand, theoretically modelling a highly complex system of coupled integro-differential equations to be solved numerically, and basing reliable predictions on such an undertaking is a task that is bound to encounter insurmountable pitfalls. So far atmospheric models have not been able to replicate the increasing number of very diverse observations that are gradually becoming available, partly as direct observations that more and more rely on satellite measurements, partly as proxy measurements.
The present paper combines state-of-the-art physics (quantum mechanics, statistical mechanics, thermodynamics, molecular spectroscopy, spectroscopic line shape analysis) with the best observations available (balloon measurements of atmospheric temperatures, infrared fluxes at the top of the atmosphere observed by satellite, detailed spectroscopic analysis of relevant molecular gases) and tries to combine the two into a consistent framework. Such an approach is what physics is all about — try to make sense of the observations, and develop a model that is capable of reproducing all the measurements. As the results of this research will show, this approach has proved to be eminently feasible.
Of course, as often in physics, there are assumptions to keep the problems tractable. Good physics is based on realistic assumptions that focus on the main issues of the problem and that remove noise from the essential considerations. In the present paper the authors neglect scattering, and do not model horizontal heat transfer via atmospheric and oceanic processes. Also, convection processes that move water vapour to higher latitudes, where latent heat is released, are not modelled as such. Of course they realize that such processes are extremely important, but representative measurements taken at different latitudes take such effects into account. It is the close connection between observations and interpretation that makes this paper very worthwhile.
The main concern of the paper is radiative transfer through an atmosphere consisting of different layers, with different temperatures and different densities of the five most important greenhouse gases. The surface of the planet is heated by solar visible radiation, and is cooled by infrared emission with a frequency distribution dictated by the Planck law. In the troposphere and part of the stratosphere the gas density is relatively high, and collisional deactivation of rotationally and vibrationally activated species dominates by far over radiative decay. In the mesosphere the lower densities allow radiative decay into space, and hence cooling. At lower altitudes greenhouse species, excited by Planck radiation from the surface, transfer their excess energy mostly to the bath of oxygen and nitrogen diatomics as rotational and translational energy. This heat is then further dissipated in the atmosphere.
In order to describe radiative transfer, the Schwartzschild Equation is the key to understanding such processes. A distinction is made between upward and downward energy flux, whose energy difference at the top of the atmosphere is lost via infrared spontaneous emission and leads to cooling.
The greenhouse gases studied have very rich spectra in the infrared region. Via the very extensive HITRAN database the frequencies and intensities of transitions are available. More than 300 000 transitions have been employed. From these observation-based intensities electric dipole transition matrix elements were derived, and hence experimental molecular infrared cross sections were obtained.
From the warm surface of the Earth, infrared radiation is emitted and eventually absorbed and transferred in the form of heat and infrared radiation to the upper layers of the atmosphere. From there this heat is lost to outer space via infrared radiation. The question is to what extent higher concentrations of greenhouse gases affect this process. In view of the CO2 debate that is raging in science and even more in politics, a sensible answer to this question is much wished for. The answer of Van Wijngaarden and Happer is clear. Doubling the CO2 concentration increases the infrared absorption by only a few percent. The reason is that extensive saturation takes place. In simple terms, if the available CO2 already absorbs almost all of the infrared energy, adding more will make little difference.
As always in physics, the proof of the pudding is in the eating. The vertical infrared intensities measured at the top of the atmosphere with a satellite-based Michelson interferometer at latitudes of the Sahara desert, the Mediterranean, and Antarctica can hardly be distinguished from the observation-based calculations produced by the authors. The agreement is excellent by any standard. Moreover, the greenhouse saturation effect is very significant, and reduces the climate sensitivity of greenhouse gases to levels much lower than suggested from theoretical climate modelling. These findings should be discussed widely and should be taken very seriously by policy makers all over the world. In summary, a landmark paper that should be published as it is, and be widely disseminated.
Prof. Dr. C.A. de Lange
Amsterdam